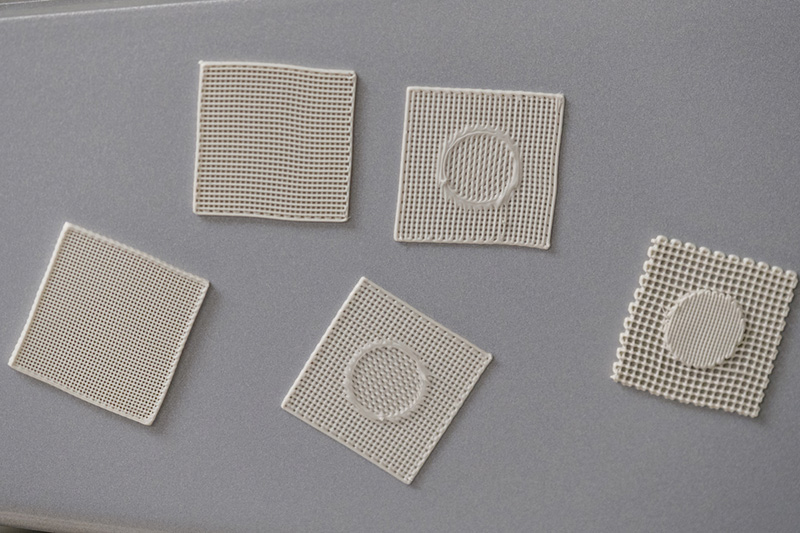
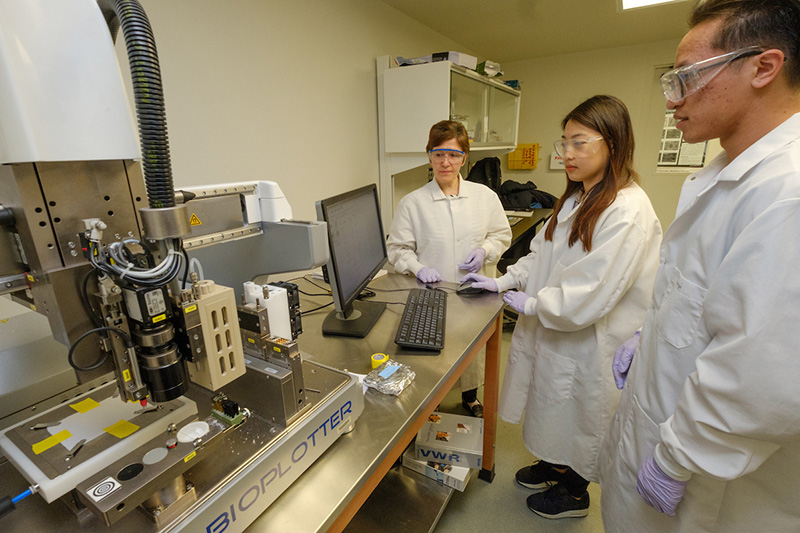
Grow-your-own bone a future goal for Miami researchers
By Andy Faught for Miami University
Growing new bone in place of ones damaged by trauma or disease may sound like the stuff of science fiction, but an interdisciplinary team of Miami researchers is harnessing technology and medical innovations to bring the idea closer to reality.
Using what’s called bone scaffolding, a lattice created with a 3-D bioplotter – an “additive” manufacturing tool similar to a 3-D printer – Amy Yousefi, professor in chemical, paper and biomedical engineering, is creating hollow polymer and ceramic bone simulations that doctors might one day seed with human stem cells or bone cells that could grow into a replacement.
“We can look at a scaffold as a temporary mechanical support where the cells can attach and eventually grow to form bone tissue,” says Yousefi, who has been working on the project since 2009. “Basically, you put the scaffolding inside the bone defect.”
Besides testing varying scaffold composites, Yousefi and students are also considering surface topographies, or roughness, to better foster cell growth. The team also is considering how porous the scaffold should be, since cells need to be able to penetrate the lattice to grow.
Colleague Jing Zhang, associate professor of statistics, models factors including the diameter of the macropores generated by 3-D bioplotters, the size of micropores, temperature in which to create them, and quantity of ceramic nanoparticles.
Joe Powers, third-year graduate student in cellular, molecular and structural biology, left, learns about the new bioreactor from Paul James. (Photos by Scott Kissell)
The more porosity, meaning the larger the holes are in the lattice, the better the chance that cells will propagate. But a larger diameter also reduces the compressive strength and could cause the scaffold to collapse. Thus far the scaffolds that Yousefi is creating in her lab are not meant to be load-bearing.
“There’s a balance between the porosity of the scaffold and the mechanical properties, and we’re looking for that balance,” she says, noting that research is still in the early stages. “If you increase the porosity to have more cells going in, you’re compromising on mechanical properties. So there’s a tradeoff. Several chemical and biological factors also affect the outcome of bone regeneration, so no one knows what the optimal scaffold should be.”
Which is precisely the goal of Yousefi’s lab.
“We’re also altering the size of the pores inside the lattice,” adds Paul James, an associate professor of biology whose specialty is molecular physiology. “There are pores that allow for the exchange of nutrients and gases so the cells are happy. That way they can access all of the things they need to stay alive.”
Larger pores are intended to facilitate the flow of oxygen and new nutrients to the cells, and they allow for easier disposal of metabolic waste, potentially toxic substances left over from cellular respiration. What is known is that macropores should have a diameter greater than 0.3 millimeters, which allows blood vessels to form and deliver oxygen to cells within the scaffold.
A bioplotter creates 3-D scaffolding samples as part of the process to try to grow replacement bones.
Currently, James is working with scaffolding that’s slightly bigger than the eraser on a pencil. On it he applies 700,000 cells.
The research is being funded by a $380,000 grant from the National Institutes of Health.
The research group recently acquired a bioreactor, an apparatus that mimics to some extent the inside of an animal, such as a human. The team will use the equipment to stress scaffolds while they are culturing cells. It’s known that exercise encourages bone cell growth in patients who have undergone bone grafts.
Scaffolds are designed to degrade as bone regenerates. They can be made to last anywhere from one month to two years.
While bone trauma typically has been addressed with bone grafted from elsewhere in the body, the trend now is to use bone substitutes, including such materials as hydroxyapatite, a mineral that is the main inorganic component in tooth enamel and bone. Substitutes are often considered easy to use and cost-effective.
Every year in the United States, Yousefi says, there are more than 1 million surgical procedures involving the partial removal of bone, bone grafting and fracture repair.
Four graduate students and several undergraduate students from engineering and biology have been working on the project. Helping in the effort is Katie Wood, a sophomore bioengineering major from Columbus. As a high school junior, she watched a TED talk on bioprinting and subsequently read Yousefi’s academic journal articles on scaffolding.
Her ambition is to run her own research lab.
“I decided that tissue engineering was what I really wanted to study,” says Wood, who uses the bioplotter to create research scaffolds. “I believe in my lifetime that doctors will be able to print a new bone scaffold, or whatever is needed to treat the patient. It’s so exciting that we are doing research that will have life-changing implications for patients in the future.”